1. Abstract
Florida depends on the oceans, yet its waters have not been extensively mapped to the highest standards. While there is a need for marine spatial data for a wide range of applications and issues, there is also a need to develop data acquisition, processing, and analytical workflows and to integrate different surveying instruments that can capture the complex and extensive coastal environment – both above and below the waterline. This note provides an overview of the research performed by scientists at the School of Forest, Fisheries, and Geomatics Sciences, University of Florida, in the field of hydrography and marine geomatics.
2. Background
The State of Florida’s coastal waters are the most valuable in the United States, and many have highlighted the need to secure and expand Florida’s Blue Economy. However, the human and natural infrastructures that sustain it are at risk (FOA, 2020). Water quality is declining (e.g., Brand & Compton, 2007), natural habitats are being damaged or lost (Parkinson & Wdowinski, 2021), and coastal infrastructure and communities are increasingly vulnerable to the growing occurrences of bigger and more frequent extreme weather events (Gao et al. 2012). Within this context, there is a critical need for geospatial and hydrospatial (Hains et al., 2021) data that span the land-water interface in Florida to produce maps to understand natural resource distribution, examine sea-level rise indicators, and inform decision-making in contexts such as coastal zone management, navigation, and coastal resilience planning.
Researchers in the School of Forest, Fisheries, and Geomatics Sciences (SFFGS, Figure 1) at the University of Florida and their partners are at the forefront of the efforts to address the challenges faced by Florida’s residents and natural systems. Our three programmatic areas, which focus on forestry, fisheries and aquatic sciences, and geomatics, allow our scientists and extension agents – who transfer scientific knowledge and expertise to the public – to adopt a multidisciplinary and sometimes interdisciplinary perspective to address these challenges.
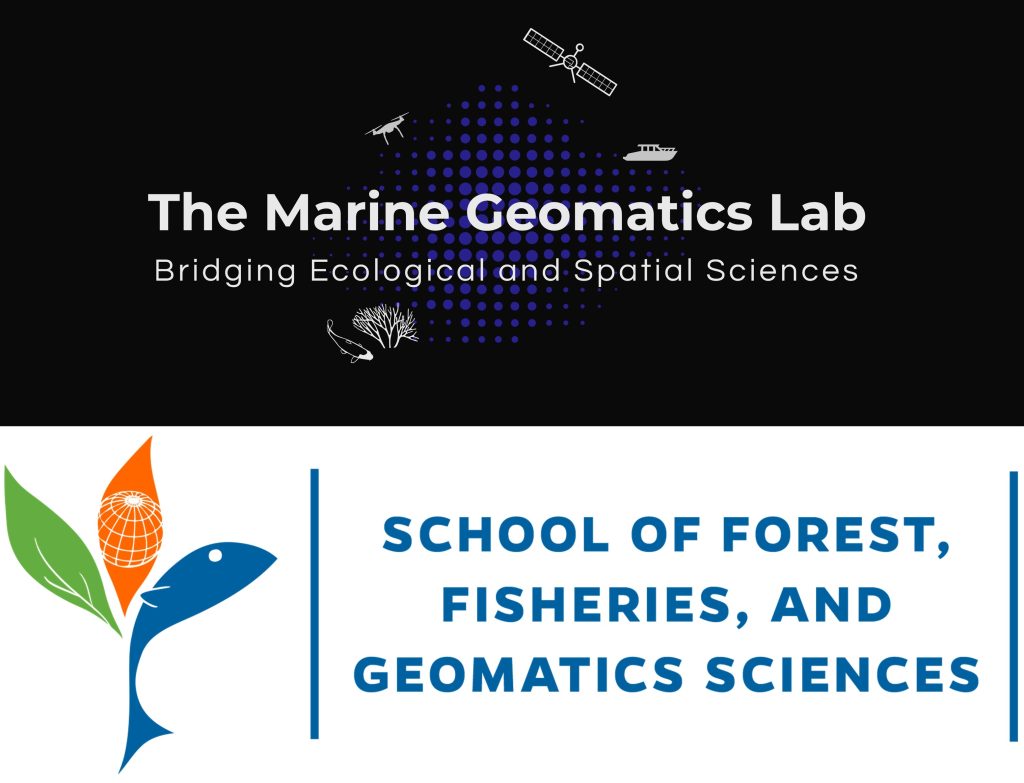
within an ecological context.
In this note and through our work, we adopt the ISO/TC 211 definition of geomatics, meaning that we consider it as the “discipline concerned with the collection, distribution, storage, analysis, processing, and presentation of geographic data or geographic information.” Our projects most often deal with one or many of the components of geomatics, and when mapping the seafloor, our work is often grounded in principles of hydrography. In fact, the “Marine Geomatics” course that we teach at the undergraduate and graduate levels is anchored in hydrography as we introduce students to the technologies, concepts, and methods required to acquire, analyze, and manage spatial data used in seafloor mapping and imaging. Course content includes instruments and plat- forms for ocean mapping, multisensor integration, positioning, hydrographic survey design and standards like S-44 and S-100, and acoustic data processing, interpretation, and management. Other courses within our curriculum introduce students to more applied contexts such as marine habitat mapping that make use of marine spatial data, including hydrographic data. This note provides an overview of how SFFGS works to address Florida’s marine spatial data needs and related applications (Figure 2).
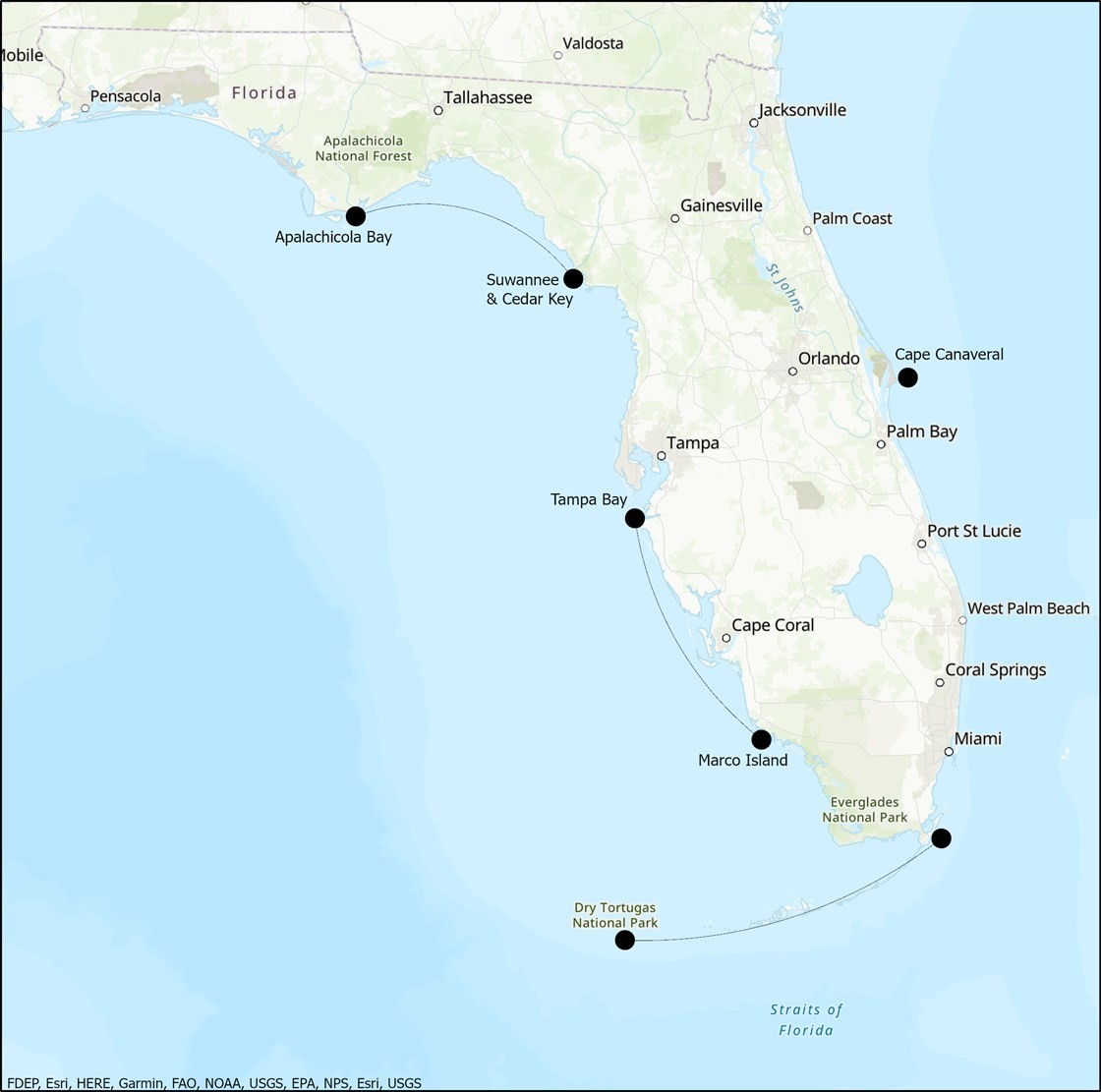
3. A Changing Climate
The Florida coast has the highest recreational use (e.g., tourism, fisheries) and has one of the highest concentrations of coastal communities in the United States. The Intergovernmental Panel on Climate Change (IPCC) estimates that coastal communities will be particularly affected by climate change, in particular due to sea-level rise, more frequent heavy precipitation events, fishery declines, water pollution, and habitat loss (Cheung et al., 2009; Bender et al., 2010; Tobey et al., 2010; IPCC, 2012). Our School has been working towards documenting the effects of climate change on Florida and predicting its impacts. We are also teaching our students, tomorrow ’s surveyors, how to combine traditional surveying with hydrographic surveying to inform sustainable coastal development in the context of predicted sea-level rise.
Through sea-level rise and increased storm activity, climate change increases coastal erosion. The Florida Department of Environmental Protection estimates that more than half of the state ’s sandy shorelines are critically eroded, threatening development, recreational, cultural, and environmental interests (FDEP, 2020). A commonly-used method to restore eroded beaches is to per- form beach nourishment through offshore dredging. However, the impacts of dredging on seafloor geo- and ecosystems are relatively unknown. The Bureau of Ocean Energy Management (BOEM) awarded a significant grant to SFFGS’s Dr. Debra Murie and colleagues to quantify the potential impacts of dredging on seafloor environments and biological communities off Cape Canaveral. This involved collecting multibeam bathymetric and backscatter data over dredged and control areas, before and after dredge events, over multiple years. The hydrographic data were combined with oceanographic data, sediment samples, biological observations, and acoustic telemetry data, among other data types, to provide a comprehensive understanding of the seafloor habitats and how they changed over time and dredging events. BOEM is still reviewing the outcomes of this long-term study. However, it demonstrated that hydrography and its concepts, like spatially-explicit vertical uncertainty, play a critical role in assessing changes in seafloor conditions through time and highlighted how seafloor morphology is directly linked to other components of the environment like hydrodynamics and biological communities.
Hydrography, marine geomatics, and the hydrospatial domain do not only help study the effects of current climate change, but can also provide insights into changes that have occurred in the past. Towards the end of the last ice age, the Gulf of Mexico coastline of Florida was roughly 200 km seaward from where it is today, and by the beginning of the most recent interval of marine transgression, about 5,000 years ago, the shoreline would have been located about 25 km away from the current day coast. Archaeological sites found along the modern-day coast of Florida suggest that people may have lived along the former shores of Florida, which are now submerged. Funded by the National Oceanic and Atmospheric Administration (NOAA) Office of Ocean Exploration and Research, we have been working with a team of anthropologists and underwater archaeologists to find evidence of cultural heritage sites along the Paleo-Suwannee River channel and tidal flats, which were first located through satellite imagery (Figure 3). This past fall, we used a small autonomous surface vehicle (Figure 4) equipped with an EdgeTech 2205 sonar to collect bathymetric, backscatter, and sidescan sonar data in the area. The data are currently being processed in collaboration with Dr. Anand Hiroji’s team at The University of Southern Mississippi and will be used to identify targets for a team of underwater archaeologists to investigate. In line with the “collect once and use many times” spirit that should guide most seafloor mapping efforts, the data will be used to produce geomorphological maps of the area. Combining them with georeferenced underwater video data from which we can identify species and substrate types will also facilitate the production of species distribution models. Since water was a constraining factor during the Pleistocene (Thulman, 2009), surveying waterways that served as conduits for travel, provided navigational waypoints, and provided habitats for large and small game resources is critical to identifying cultural heritage sites (Dunbar, 2016). With these waterways now submerged, hydrography is necessary to support research on how peoples responded to social and environmental factors and mitigated the effects of past sea-level rise, which could contribute to current resilience efforts in the face of modern-day sea-level rise (Cooper & Peros, 2010).
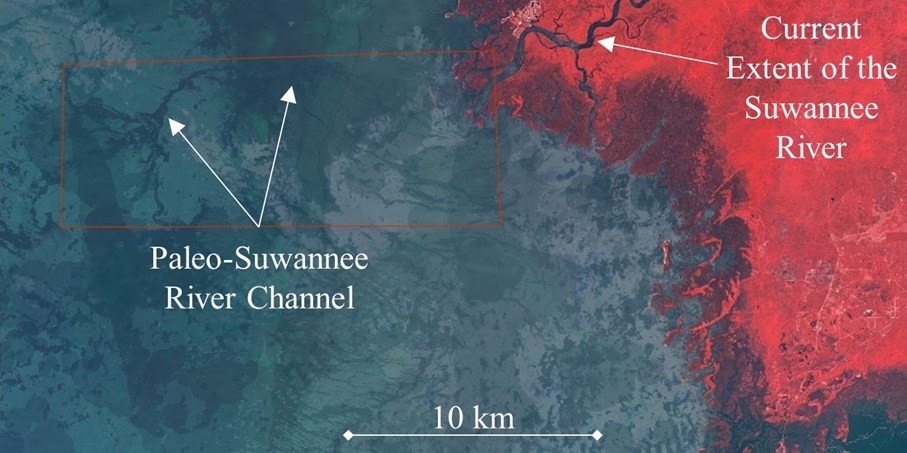
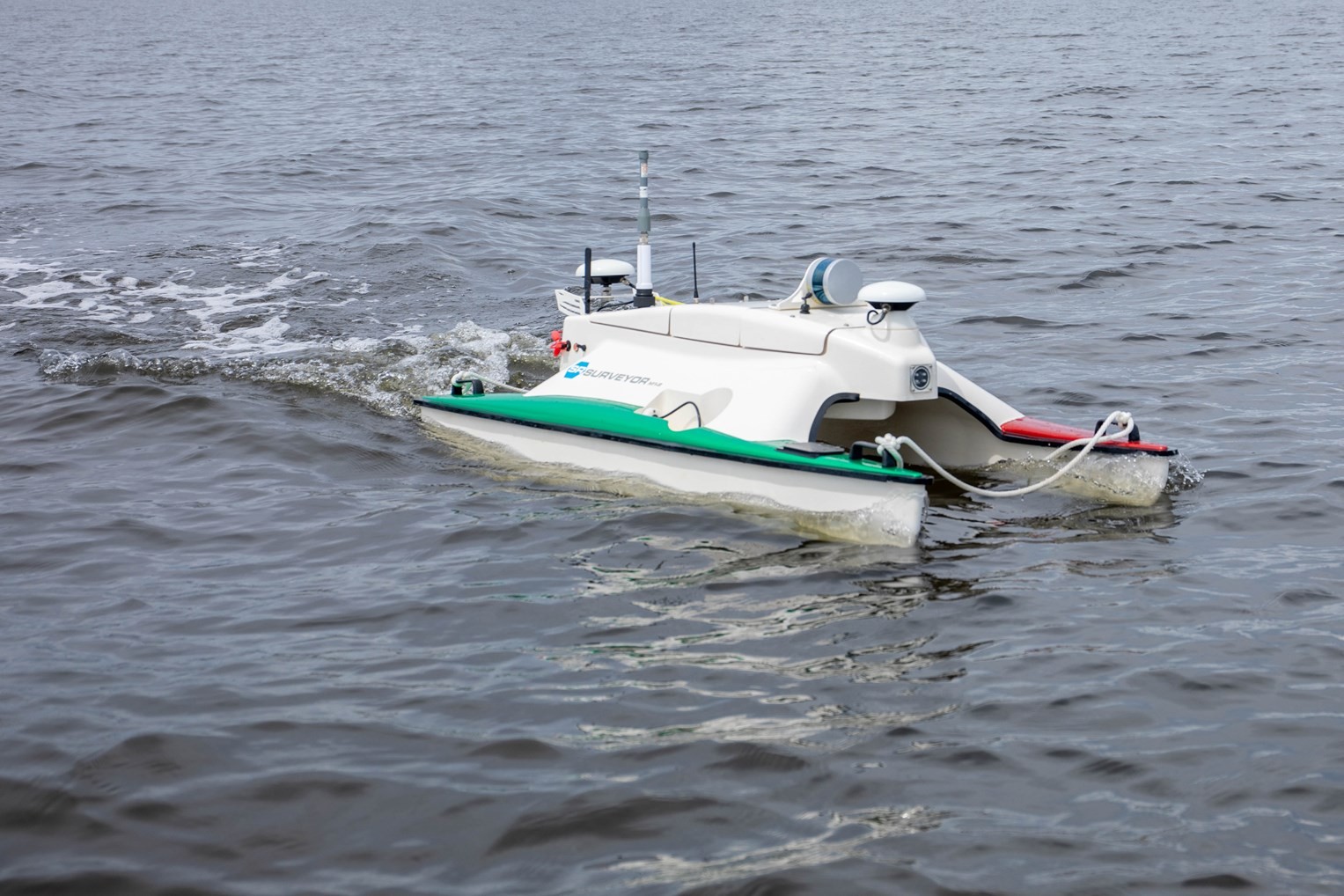
4. Producing Bathymetric and Coastal Elevation Models
In recent years, satellite-derived bathymetry (SDB) has gained significant traction as a relatively low-cost, broad-coverage, and high temporal resolution means to produce bathymetric data in coastal areas. Our program is involved with the Satellite-Derived Bathymetry Best Practice Project Team (SDB-PT) of the International Hydrographic Organization Hydrographic Surveys Working Group (IHO HSWG), an international effort to identify best practices in satellite-derived bathymetry in line with the S-44 Standards for Hydrographic Surveys. In parallel to these efforts, we also contribute to advances in satellite-derived bathymetry methods and applications. For example, we developed a framework for SDB analysis applicable to large diversified areas using multi-date Sentinel-2 satellite imagery and regression-based random forest analysis. We tested our approach in the nearshore along more than 200 km of coast in southwest Florida. Model calibration and validation were done using airborne lidar bathymetric data. Combining the spectral bands of multiple historical satellite images yielded the highest accuracy, with root mean square error values of 8% in waters shallower than 13.5 m, which corresponds to the depth penetration limit of the lidar surveys used. Since the quality of the satellite imagery is a significant factor influencing the accuracy of the bathymetry estimation, we developed a workflow to optimize the selection of Sentinel-2 images to incorporate in the analysis. In terms of applications, we often integrate SDB within our other projects. For example, we are exploring using the acoustic data collected for the underwater archaeology project described above as training and validation data for producing a broader-scale bathymetric model of the area from satellite imagery. Akin to what has been done in land-based archaeology (e.g., Chase et al., 2012; Jones et al., 2019), the potential of the produced satellite-derived bathymetry to serve as proxies of sites of cultural heritage will then be tested.
When studying coastal regions, it is often necessary to combine hydrographic data with terrestrial elevation data to get a more complete understanding of complex and dynamic environmental patterns and processes. We have collected a huge amount of unoccupied aerial systems (UAS) geospatial data in almost 50 field campaigns since 2019 in support of geomatics research and ecological monitoring efforts along the Florida coast, partnering with universities and local, state, and federal entities. The UAS imagery and laser scanning data collected represent fine-scale snapshots of a wide variety of coastal ecosystems that can be used to document and model processes occurring due to climate change, sea-level rise, and storm events. In addition to collaborative efforts, our group has independently investigated and developed best practices for UAS data collection and calibration, uncertainty estimation, and machine learning approaches to extract highly accurate critical information from the raw data. In particular, we have investigated methods for precise UAS lidar adjustment, accuracy assessment, range bias mitigation, and the challenging task of bare earth filtering for vegetated coastal areas (e.g., Pinton et al., 2020, 2021), all crucial for rigorous modeling of fine-scale coastal changes.
5. Monitoring Coastal Habitats
Coastal habitats in Florida provide a wide range of critical ecosystem services, for example by protecting from erosion and storms, providing opportunities for tourism and outdoor activities, providing habitats for other species, and enhancing local fisheries. Many of these habitats and the services they provide are facing extreme pressure, such as unsustainable tourism and coastal development, inadequate protection and management, and pollution. Ongoing restoration and monitoring efforts often do not have baseline data against which to quantify success or failure. There is therefore a need for frequent, effective, and comprehensive mapping and monitoring methods, which marine geomatics can provide. One of the primary challenges when trying to monitor intertidal habitats is that sometimes they are under the waterline, and sometimes they are above the waterline, meaning that multiple approaches must be combined to provide a comprehensive understanding. Our program has contributed to statewide efforts to develop monitoring approaches that combine optical and acoustic remote sensing for coastal habitat monitoring in the past few years.
We have studied many estuaries and creeks off an area of the west coast of Florida called the Nature Coast, off the towns of Cedar Key and Suwannee. A combination of intertidal oyster reefs, salt marshes, and mudflats is found in these areas (Figure 5). The different habitat types form a dynamic ecosystem in which unhealthy oyster reefs can transform into mudflats that salt marshes can then populate, and accurate temporal monitoring is critical to capture these subtle changes. Our work combines UAS-derived multispectral imagery (up to ten spectral bands), structure-from- motion (SfM) photogrammetry digital surface and terrain models (DSMs and DTMs), and lidar data. So far, we have focused on object-based image analysis for identifying and delineating habitat types (e.g., Espriella et al., 2020) and on understanding the topographic structure of the different habitat types using geomorphometry (e.g., Lecours & Espriella, 2020, Figures 6 and 7). Our current semi-automated classification efforts reach accuracies in the low 90%, and the integration of topographic and bathymetric information is likely to improve these even more. The use of DSMs and DTMs is critical to these efforts as the spectral signature of the different habitat types can often be similar and vary with wetness levels (e.g., if the tide just retreated). We also demonstrated that the vertical structure and complexity of intertidal oyster reefs are linked to their health, which highlights the importance of collecting topographic and bathymetric data. In order to test whether what we observed in intertidal reefs is also true in subtidal reefs (i.e., those that are permanently submerged), we have mapped and imaged the Suwannee Reef, an important yet potentially declining reef complex on the west coast of Florida, with an EdgeTech 2205 mounted on a small autonomous surface vehicle (Figure 2). These hydrographic surveys will provide baseline data to state partners and contribute to their monitoring efforts while allowing us to test our hypotheses related to the topographic/bathymetric structure of the reefs. In April 2022, we plan to collect underwater lidar, apply SfM photogrammetry techniques on underwater videos, and apply SDB methodologies on UAS multispectral data to produce UAS-derived bathymetry. These parallel data collection efforts will allow us to directly compare fine-scale bathymetric measurements in a structurally complex environment from many different technologies.
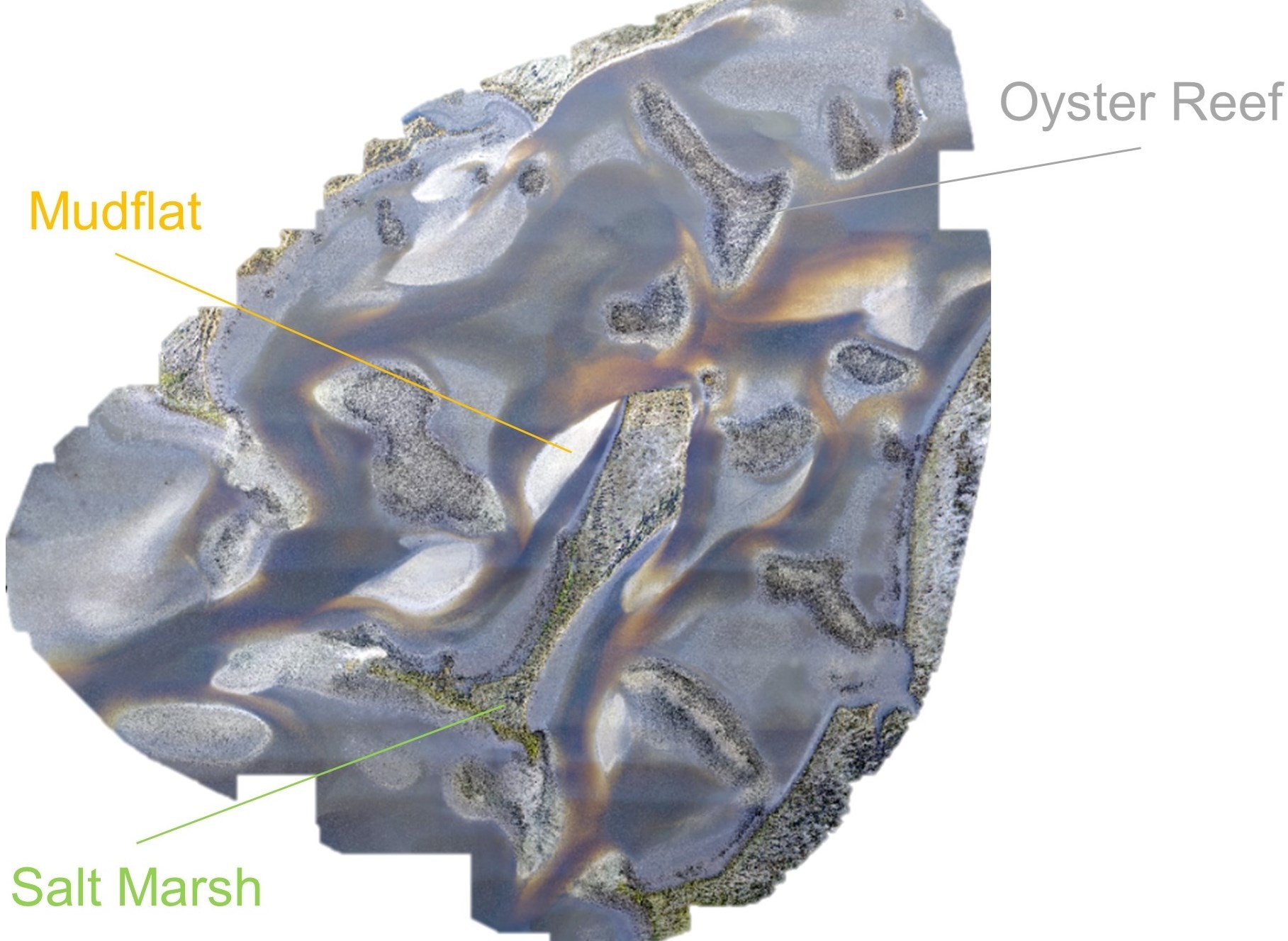
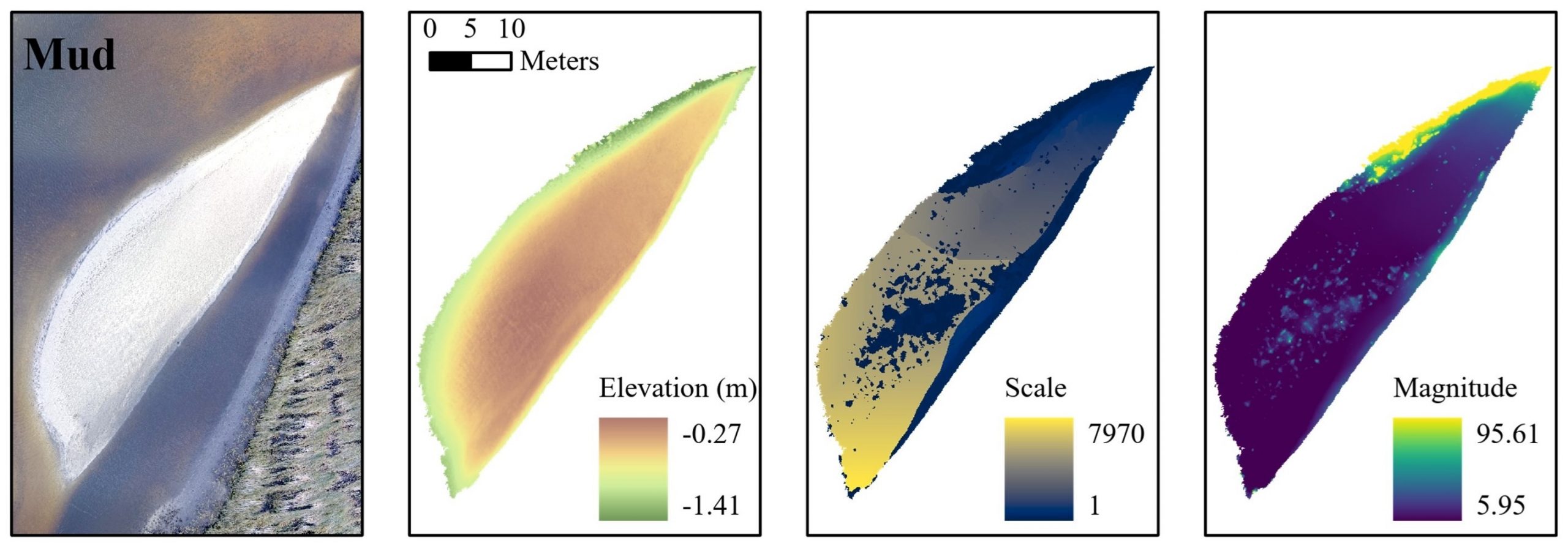
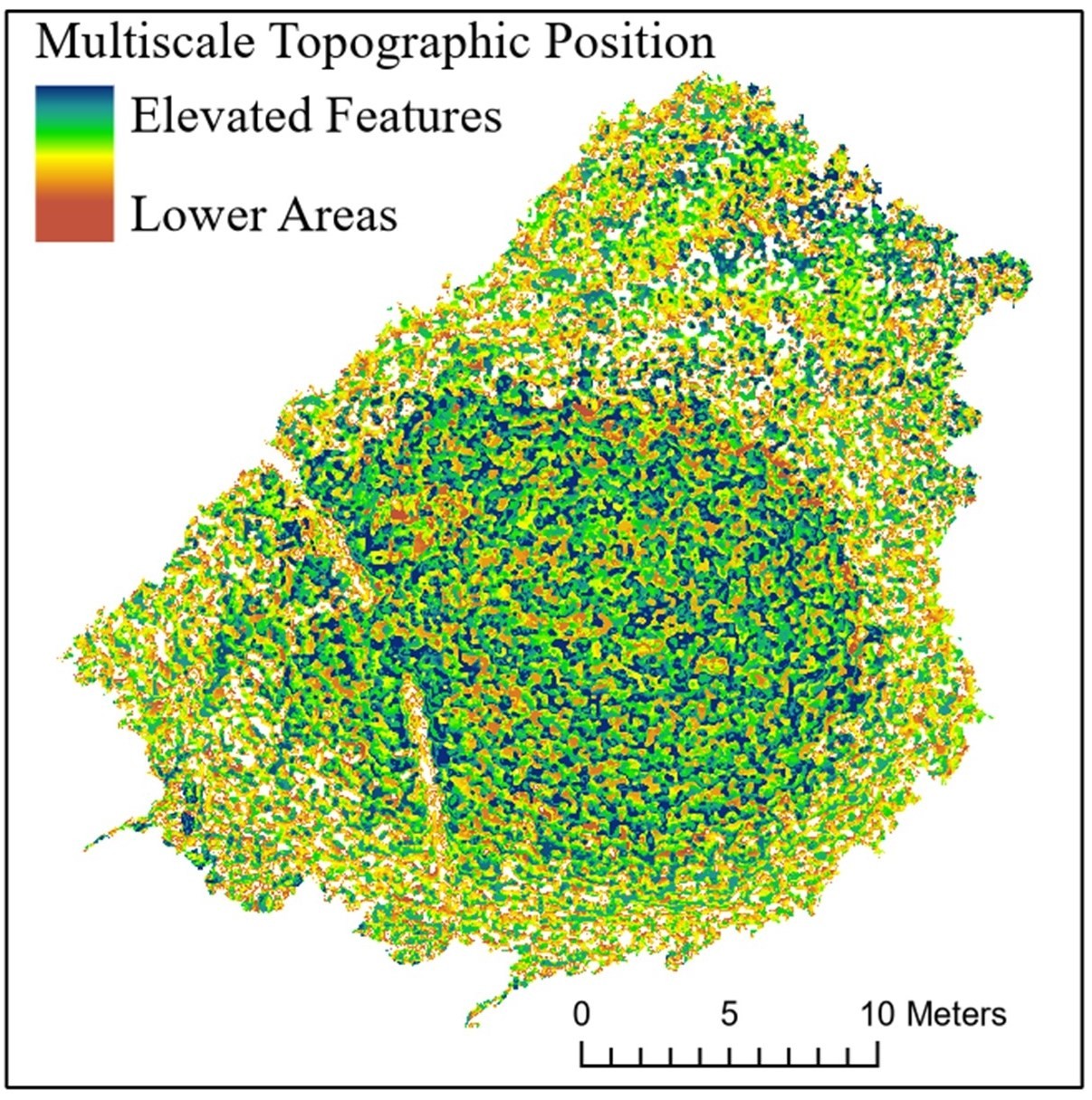
While most of our methodological developments occur while studying oyster reefs – due to their relative proximity to our campus, many of the workflows we develop are transferable to other habitat-forming species, such as coral reefs. In collaboration with the Florida Fish and Wildlife Conservation Commission’s (FWC) Coral Reef Evaluation and Monitoring Program, our team is investigating the use of SfM photogrammetric reconstruction of coral reefs from historical aerial imagery. The produced DSMs are then used to derive complexity metrics that relate to coral colony success. The general goal of this work is to fate-track coral colonies through historical imagery to characterize survivorship and fine-scale topography in chronically disturbed coral reefs in the Dry Tortugas National Park while also demonstrating the feasibility of using geomatics tools for answering regional-scale ecological questions.
6. A Standardized Mapping Framework
While there has recently been an increase in seafloor mapping efforts in Florida by governments, academic, and non-profit organizations, there is currently no standardized framework for marine spatial data and metadata integration and distribution. In 2020, the Florida RESTORE Act Centers of Excellence Program, managed by the Florida Institute of Oceanography, funded the establishment of the West Florida Shelf Standardized Mapping Framework Center of Excellence in our School. The purpose of this effort is to build a process for and get community agreement on a framework to support effective and dynamic aggregation of current and future seafloor mapping data. We have been reviewing existing and successful frameworks for marine data integration and distribution around the world and existing standards, protocols, and guidelines for marine data collection, integration, and distribution. While standards for hydrographic data and metadata have been set and are updated periodically at the international and national levels, standards for other data types like sediment samples or ocean chemistry data are not as widely adopted. Our work should provide a framework to ensure that all Florida marine geospatial data are digitally resilient, i.e., accessible, interchangeable, operational, and of reasonable quality for given uses, thus ensuring that data can be used many times for different applications.
7. Conclusion
Hydrography is an inherent component of marine geomatics and hydrospatial. Without hydrographic data, we could not understand the primary characteristics of marine habitats, identify navigational hazards following hurricanes, or assess coastal vulnerability and resilience. While this note provides an overview of our group’s work in marine geomatics, it is important to recognize the contribution of many other groups that contribute to understanding Florida ’s coastal waters using hydrography and marine geomatics, including different groups at the University of Florida, the Florida Institute of Oceanography, the Florida Coastal Mapping Program, the Center for Ocean Mapping and Innovative Technologies at the University of South Florida, NOAA ’s Mission: Iconic Reef, FWC, and many other academic, governmental, and non-governmental organizations. Together, we grow the potential of marine and coastal spatial data to contribute to decision -making in contexts such as coastal zone management, ecosystem restoration, navigation, and coastal resilience planning.
8. References
– Brand, L.E. and Compton, A. (2007). “Long-term increase in Karenia brevis abundance along the Southwest Florida Coast”, Harmful Algae, 6, pp. 232-252.
– Chase, A.F., Chase, D.Z., Fisher, C.T., Leisz, S.J. and Weishampel, J.F. (2012). “Geospatial revolution and remote sensing LiDAR in Mesoamerican archaeology”, Proceedings of the National Academy of Sciences of the United States of America, 109, pp. 12916-12921.
– Cheung, W.W.L., Lam, V.W.Y., Sarmiento, J.L., Kearney, K., Watson, R., and Pauly, D. (2009). “Projecting global marine biodiversity impacts under climate change scenarios”, Fish and Fisheries, 10, pp. 235-251.
– Cooper, J. and Peros, M. (2010). “The archaeology of climate change in the Caribbean”, Journal of Archaeological Science, 37, pp. 1226-1232.
– Dunbar, J.S. (2016), Paleoindian Societies of the Coastal Southeast, University Press of Florida, Gainesville, FL.
– Espriella, M.C., Lecours, V., Frederick, P.C., Camp, E.V. and Wilkinson, B. (2020). “Quantifying intertidal habitat relative coverage in a Florida estuary using UAS imagery and GEOBIA”, Remote Sensing, 12, pp. 1-17.
– FDEP (2020). Why restore eroded beaches?, Florida Department of Environmental Protection, viewed 24 February 2022, https://floridadep.gov/rcp/beaches-funding-program/content/why-beach-restoration.
– FOA (2020). Securing Florida’s Blue Economy: Stategic policy plan for Florida’s oceans and coasts. Florida Ocean Alliance. 120 p.
– Gao, Y., Fu, J.S., Drake, J.B., Liu, Y. and Lamarque, J.-F. (2012). “Projected changes of extreme weather events in the eastern United States based on a high resolution climate modeling system”, Environmental Research Letters, 7, pp. 1-12.
– Hains, D., Ponce R., Oei, P., Gülher, E., Pang, P.Y., Cove, K., Cawthra, H.C., Bergmann, M. and Picard, K. (2021). “So… what is hydrospatial?”, International Hydrographic Review, 25, pp. 115-122.
– IPCC (2012), Managing the risks of extreme events and disasters to advance climate change adaptation, Cambridge University Press, New York, NY.
– Jones, P.J., Williamson, G.J., Bowman, D.M.J.S. and Lefroy, E.C. (2019). “Mapping Tasmania’s cultural landscapes: using habitat suitability modelling of archaeology sites as a landscape history tool”, Journal of Biogeography, 46, pp. 2570-2582.
– Lecours, V. and Espriella M. (2020). “Can multiscale roughness help computer-assisted identification of coastal habitats in Florida?” In: Alvioli M, Marchesini I, Melelli L & Guth P (eds), Proceedings of the Geomorphometry 2020 Conference, pp. 111-114.
– Lindsay, J.B., Newman, D.R., and Francioni, A. (2019). “Scale-optimized surface roughness for topographic analysis”, Geosciences, 9, 322.
– Newman, D.R., Lindsay, J.B., and Cockburn, J.M.H. (2018). “Evaluating metrics of local topographic position for multiscale geomorphometric analysis”, Geomorphology, 312, pp. 40-50.
– Parkinson, R. and Wdowinski, S. (2021). “Accelerating sea level rise and the fate of South Florida coastal wetlands”, SSRN, pp. 1 -37.
– Pinton, D., Canestrelli, A., Wilkinson, B., Ifju, P. and Ortega, A. (2020). “A new algorithm for estimating ground elevation and vegetation characteristics in coastal salt marshes from high- resolution UAV-based LiDAR point clouds”, Earth Surface Processes and Landforms, 45, pp. 3687-3701.
– Pinton, D., Canestrelli, A., Wilkinson, B., Ifju, P. and Ortega, A. (2021). “Estimating ground elevation and vegetation characteristics in coastal salt marshes using UAV-based LiDAR and digital aerial photogrammetry”, Remote Sensing, 13, pp. 1-30.
– Thulman, D.K. (2009). “Freshwater availability as the constraining factor in the Middle Paleoindian occupation of North-Central Florida”, Geoarchaeology, 24, pp. 243–276.
– Tobey, J., Rubinoff, P., Robadue Jr., D., Ricci, G., Volk, R., Furlow, J. and Anderson, G. (2010). “Practicing coastal adaptation to climate change: lessons from integrated coastal management”, Coastal Management, 38, pp. 317-335.