Abstract
1 Introduction
Hydrography is an engineering science, which does not seek knowledge for knowledge’s sake. The goal is not to get a description of the marine topography and geophysical processes of the oceans just for pure knowledge, instead it is demand-driven. History confirms this. It was the American Benjamin Franklin who, as early as 1769, discovered through systematic observations how the force of the Gulf Stream could be useful to ships navigating between the North American continent and Europe (Fig. 1). Another American, Matthew Fontaine Maury, organized the first systematic deep-sea surveys in the Atlantic in the 1850s to prepare for the laying of undersea telephone cables.
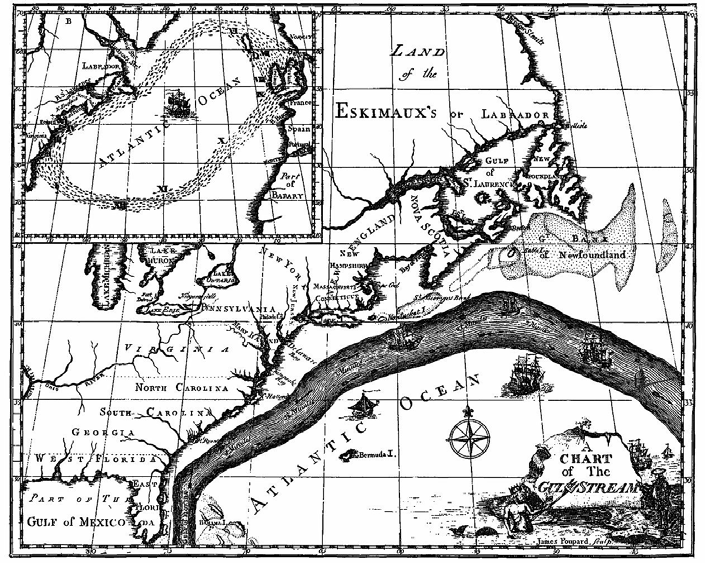
Compared to the complexity required to measure physical quantities, for example at the atomic scale, the continuous monitoring globally of water levels, current directions and velocities, as well as the measurement of underwater topography, do not appear complex tasks for modern technologies. How can it be explained then that only about 25% of the seabed has been covered by modern measuring methods so far (Fig. 2)? The challenges lie in the sheer size of the area to be measured which makes up about 70% of the Earth’s total surface, in its constant variability due to natural processes, the inaccessibility of the seabed, which reaches water depths of up to 10,000m, and the still inadequate mathematical modelling of global geophysical processes at the oceanic scale due to the lack of high-resolution data.
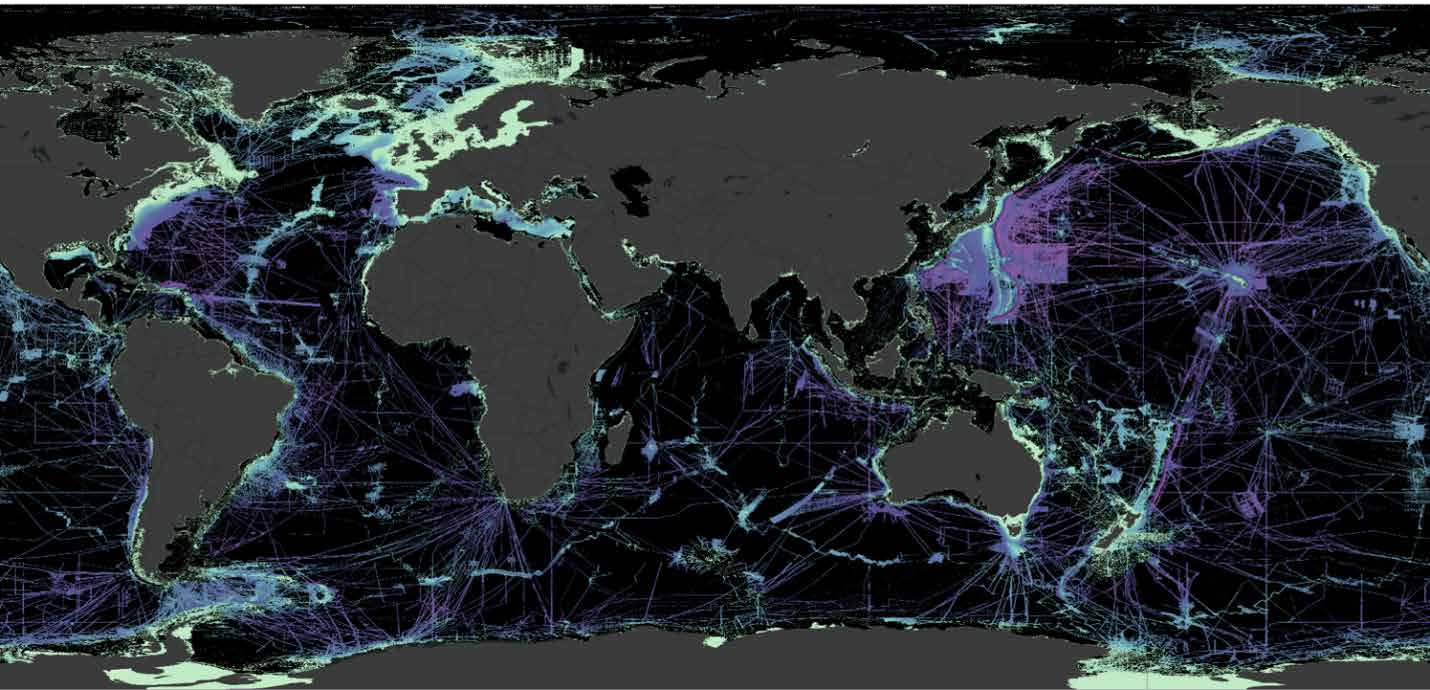
This analysis is not new and yet still highly topical, because the demand for more precise, comprehensive and up-to-date information on the physical properties of the seas and oceans far exceeds the available supply. The major need comes from all the stakeholders who deal with political, environmental and economic issues relating to the use and protection of the oceans. Since the majority of the world’s population lives on, from and with the oceans, a demand-oriented provision of hydrographic information is of fundamental importance for present and future generations. Furthermore, it should not be forgotten that the oceans are the largest weather and climate machine, whose function has a general impact on all inhabitants of the earth but more specifically on those living on small island states and along increasingly eroding coasts.
Hydrography is called upon to serve this need for information in support of informed decision-making. Starting from the present status and the discernible trends in technical development, this article aims to provide a glimpse into the expected future development up to the end of the current decade.
2 The state of the art and the need
The previously mentioned figure of 25% of submarine topography recorded in good resolution requires further explanation. The water depths up to the continental shelf, which extends to a depth of 200m, can be assumed to be well recorded and thus known with sufficient accuracy in many parts of the world. Exceptions are along some coastal areas in Africa, the Antarctic waters and the Arctic, where the dwindling sea ice is constantly revealing new, previously unsurveyed areas. The greatest gap is in the deep sea at depths beyond 200m, which should be considered as mostly unsurveyed so far (Fig. 2). Due to the comparatively low penetration depth of optical signals in the water column, only satellite altimetry provides approximate indications of the shape of underwater formations. For more precise surveys, mainly ship-based hydroacoustic deep-sea echo sounders are used, which are not very economical in terms of their operational costs, carbon footprint and area efficiency. Recent developments rely on crewless operating instrument carriers – the uncrewed surface vehicles (USV), which are confronted with the problem of the high energy requirements of low frequency deep-sea echo sounders.
In navigable shallow waters with high traffic volumes, such as estuaries and harbors, hydroacoustic techniques are used to provide high-frequency and very accurate surveying services that enable the safe and efficient passage of ships, as well as to monitor the structural condition of port facilities, bridges and dikes. So far, however, only ports in the Americas, Europe and Asia, and a few in Oceania and Africa that are important to the global economy are capable of providing such ongoing data service provisions to operators and customers.
For shallow waters with water depths of up to 30m, optical and radar-based satellite observation systems provide information on water depths and water levels which, although less accurate than on-site measurements, have advantages due to their wide aerial stretch at comparatively low costs. Their evaluation requires highly qualified algorithms cast in software and qualified personnel for their application.
Water levels and currents are recorded continuously near the coast, especially in areas with significant maritime traffic, and mostly with sufficient accuracy thanks to level measurement systems and current meters. Our knowledge of oceanic parameters such as the global distribution of water temperature and salinity has grown considerably over the past twenty years thanks to the deployment of drifting buoys through the ARGO programme. One day ARGO floats may provide depth soundings as well, however, drifters cannot replace continuous positional high-resolution observations. Certainly, there are continuous and well established global networks for sea level measurements (Global Sea Level Observing System (GLOSS) and the associated Permanent Service for Mean Sea Level (PSMSL)) in place. A permanently financed, global, fine-meshed measurement network, as is standard on land for meteorological data, however, is still lacking for physical (as well as chemical and biological) parameters of the oceans.
The development of such a perpetual measurement infrastructure requires affordable, powerful and robust sensor technology. In the development of such autonomously operating sensor technology, the energy supply and wireless transmission of the recorded measurement data pose particular challenges.
Although the aforementioned deficits exist for the long-range and permanent recording of hydrographic information, the scope of the already continuously recorded measurement data on a global scale is considerable. However, their accessibility is subject to significant restrictions of a geopolitical, scientific-political and economic nature. In a resolution endorsed by the Economic and Social Council (ECOSOC) in 2015, the UN Committee of Experts on Global Geospatial Information Management declared its support for common principles of geospatial data management, which also regulate the provision and availability of maritime geodata. Unfortunately, governmental commitment and practice in the field often do not match when it comes to hydrographic information. Existing knowledge about the topographical nature of one’s own coastal waters is rarely shared extensively for national security reasons. A similar situation can be observed for data collected within the framework of scientific projects: their preparation for public availability is costly and is usually not taken into account in project financing. The data itself often provides the basis for the scientific objectives based on it and thus justifies its exclusivity for the current project. Owners of data collected in a costly manner for private-sector purposes expect remuneration for making it generally available or are reluctant to let authorities and competitors share in their information advantage. Positive attempts to improve the situation is the digital strategy of the European Union for promoting reuse of open data codified by means of the Public Sector Information Directive and the commitment of now 33 IHO Member States to allow crowdsourced bathymetry within their waters of jurisdiction (Fig. 3).
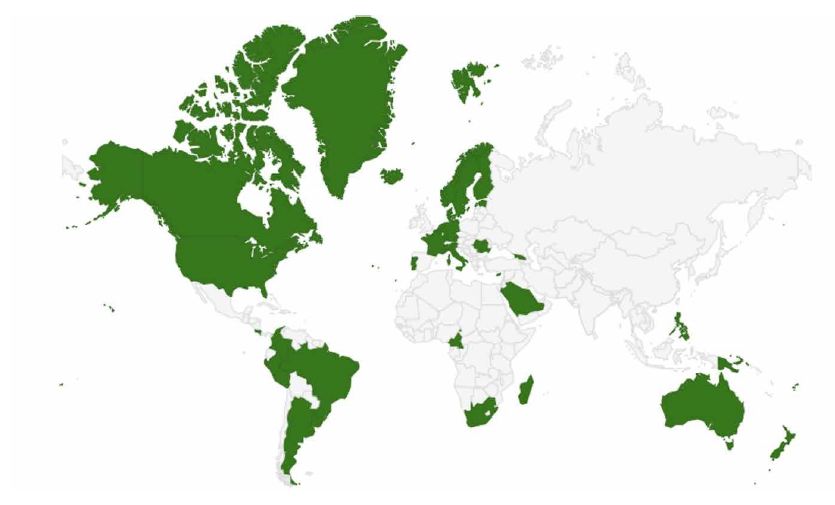
The global growth in the sheer volume of data is impressive. Hydroacoustic and satellite-based methods deliver data in enormous resolution in terabytes that grow to petabyte collections. Their transmission requires large bandwidths; the software effort, including Machine Learning and Artificial Intelligence components for their management, processing and interpretation is immense. A holistic view of the state of the ocean cannot succeed by looking at individual phenomena such as the distribution of salinity or global ocean currents in isolation. Their interpretation requires the correlation of the different themes at the data level; their evaluation requires the application of powerful statistical algorithms. In order to bring together the data from the various disciplines, comprehensive data standards are needed that are based on a common data model and that can be expanded without limiting the mutual compatibility of the data sets.
The classic task of nautical hydrography providing shipping with thematic charts for navigation is fulfilled with a worldwide stock of digital and, with a decreasing tendency, printed nautical charts. The fact that all digital navigational chart datasets (ENCs) for international shipping fully comply with the governing IHO Standard is a great achievement. The technical philosophy underlying the ECDIS system, however, dates back to the nineties of the last century. Today, land-based navigation systems show the enhanced possibilities of specialized software to support navigation, which go far beyond the ECDIS functions. They are achieved through the thematically rich data used and the integrated real-time online components. The generation of users that has grown up with the internet expects the same for ship-based navigation systems. Only with the expansion of the functional scope of a new generation of ECDIS devices can further efficiency and safety gains be realized in shipping and the associated port services. The same applies to the introduction of uncrewed, remotely or autonomously operating watercraft, which require a completely new quality of support with hydrographic information in the form of data services.
3 Expected developments
The fundamental physical principles on which hydrographic measurement systems are based can neither be extended nor overridden. Therefore, it is to be expected that the established hydroacoustic and laser-based methods for on-site measurements and the optical and radar-based methods in remote sensing will remain dominant. Quantum-based measurement methods, should they be developed, will probably not reach practical maturity in the current decade. Further improvements, however, can be expected in the resolution of sensor technology, the interpretation of measurement signals and the correlation of onsite measurements with remote sensing information through the use of artificial intelligence algorithms. Through the intelligent evaluation of hydroacoustic measurement signals, for example, new insights into the nature of the sediment, sea grass and other characteristics of the habitat can be gained if national hydrographic services will not only entitled but also enabled by resources to undertake such tasks which are currently done only erratically with research projects. In particular, the mapping of sea grass meadows as ideal CO2 reservoirs can make an important contribution to their cultivation and thus to decarbonization.
As instrument carriers, flying, floating and diving remote-controlled or autonomously operating units will become dominant because of their excellent cost/benefit ratio. This development will drive the miniaturization of sensor technology while reducing their energy consumption. This will give a further boost to the accurate measurement of coastlines and thus benefit coastal protection and land-sea transition use enormously. An important demand driver is the installation and monitoring of offshore structures in energy production, submarine cables and pipelines, as well as fish farming and other forms of protein production at sea.
In addition to the lower costs for the units carrying the measuring devices themselves, their area efficiency due to their unlimited usability in terms of time with simultaneously increased surveying speed also enables the reduction of the distance of the sensor system to the object under investigation. This makes it possible to use high-resolution laser detection at short distances, even directly in the body of water. The energy supply remains a challenging problem that can be tackled by solar foils that can be adapted to curved construction forms, thermal energy generation from the temperature differences from water layers of different depths or possibly by miniaturized fuel cells (Fig. 4). Nevertheless, uncrewed units will be the disruptive technology in the hydrographic domain – driven by the regular duties described but also by emerging new national policies and strategies on the protection of sea bed national interests (power and internet submarine cables).
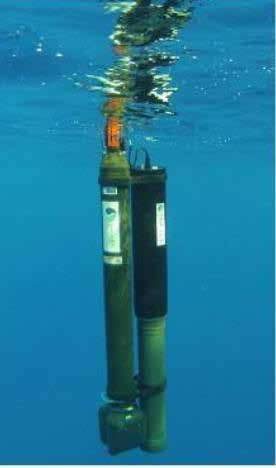
Enormous changes will be brought about by the long-announced but now imminent global availability of affordable broadband communications at sea. Although the lack of broadband underwater communication remains, hydrography will nevertheless become a communication-driven discipline. It remains to be seen whether this will break the current trend of pre-processing data on the instrument carrier with edge computing in order to reduce the amount of data to be transmitted. In any case, it will be possible to transmit sensor data in real time and to combine them in a server-based architecture into a situation picture that can be permanently retrieved by all participants. Technologies that use hydrographic cyber-threat proof information on the basis of decentralized databases in the cloud, as is the case for ECDIS with ENCs, for example, could follow the example of internet-based map services whose central database is constantly kept up to date and thus become a client-server architecture.
The trend towards digital maps is irreversible. However, similar to everyday office life, there will still be a need for a hard copy of digital chart content in various application scenarios. The automation of the main cartographic tasks – levelling, reducing and generalizing – must be made even more user-friendly in order to make the paper map printable by the customer. Generalization is the supreme discipline of cartography, even in the digital world. The nautical-hydrographic information formerly distributed in analogue form, such as nautical handbooks, current atlases and tide tables, have already been converted into digital variants without exception. However, these digital variants have so far mainly followed the printed models: The information streams are separate from each other and their presentation is two-dimensional. Examples of this are the paper-map-like presentation of ENCs in ECDIS and the so far insufficient integration of water level feeds and sea current information into this system.
The second wave of digitization of hydrographic information using the S-100 data model, which is currently being pushed by the IHO, will result in a massive expansion of digital data services that will be compatible with each other at the model level and thus interoperable. Their full potential could unfold through spatial-temporal representation. Some user groups favor the use of the term “hydrospatial” to mark a linguistic distinction from the techniques introduced so far. This argument can be followed insofar as the technique of visual two-dimensional overlaying of constantly new layers of information makes the acquisition of the actual situational relevant information more difficult rather than easier. In order not to overtax the user in his visual perception possibilities, the algorithm-based situational selection of the information to be displayed and new forms of presentation will be necessary. It is questionable whether the general reservations against such a pre-selection, which is difficult for the user to evaluate, will diminish over time. For some years now, there have been discussions about presenting hydrographic information in a way familiar to the world of computer games in three dimensions or by means of augmented reality (Fig. 5). Due to the strong regulation of nautical equipment in professional navigation and the limited market size of the entirety of all watercraft, a broad acceptance or market penetration with such technologies is difficult to predict. However, specialized applications in hydraulic engineering, dredging and offshore applications could soon benefit from these technologies.
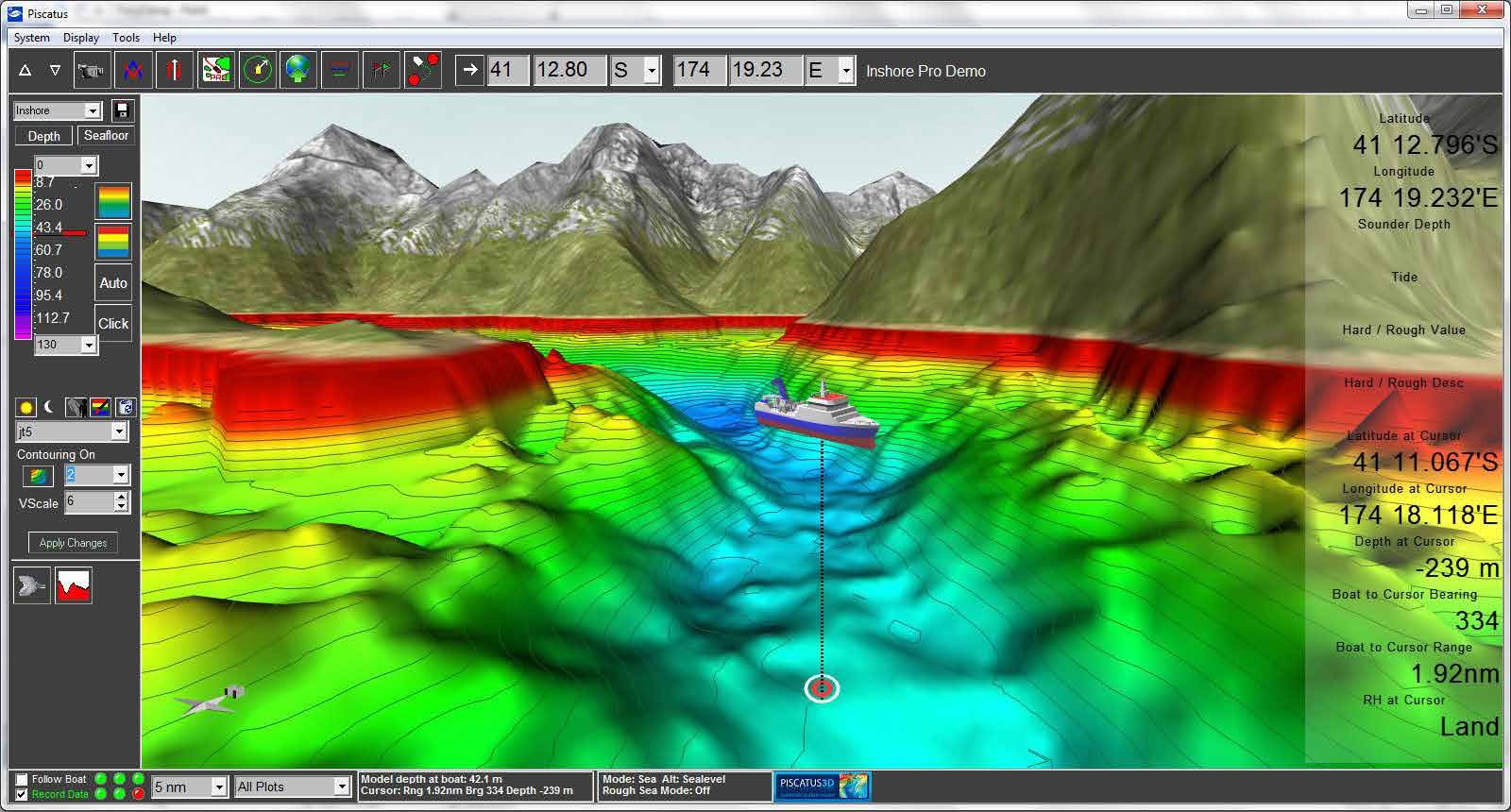
Hydrography is the provider of baseline digital information streams when it comes to providing a holistic picture of the state of the seas and oceans. The latest terminology to summarize these efforts is the digital twin of the oceans. Digital twins are virtual representations of physical objects and systems, the physical or real twin, which in this case is the ocean or a part of it. However, the generic term digital twin does not address a single system that completely represents the state of the ocean. According to the current understanding, there will be several topic-oriented twins whose practical application will take place in specially adapted geoinformation systems. Digital twins include predictive and data-driven models that users can interact with to support their needs. Digital twins thus provide the ability to make informed operational, management, and policy decisions for the real twin. This connection between the digital twin and the real one requires a well-formulated interface between the digital twin, environmental data, and the user. User interaction is therefore an essential function that is embedded in the design of digital twins, including visualization, user-driven data transformation and data-science tools.
In order to anticipate the possible lines of development of future maritime geoinformation systems, it has proven useful to compare similar development goals and already existing systems for land applications. Obvious parallels are the trend towards thematic diversity of the data to be combined with each other, the embedding of metadata as a necessary prerequisite, the motivation to expand data types while at the same time maintaining strict semantic standardization to ensure compatibility and the maintenance of loss-free communication between machines. These prerequisites are served by the already mentioned S-100 data model of the IHO (Fig. 6). Its implementation as a high priority project of the member states of the IHO in the current decade can only be considered successful if it is possible to establish regular S-100 compatible data streams for the data products covering hydrographic services’ core tasks and to motivate industry to develop attractive applications based on these data.
This requires hydrographic services to establish new types of production systems with far-reaching consequences for the technical equipment and skills of employees involved. The transition from the product-centric production of nautical documents to a data-centric production of a diversity of digital data products driven by market demand is thus finally completed. This comprehensive digitalization of the entire chain of collection, processing and dissemination of information is a major challenge in an administrative environment characterized by official procedures. Beyond the associated conversion of the overall system, a special effort must be made to define and apply quality parameter for the data sets used. The regular operation of autonomous surface ships at sea, as they are currently being defined by the IMO, is unthinkable without being able to qualify hydrographic information.
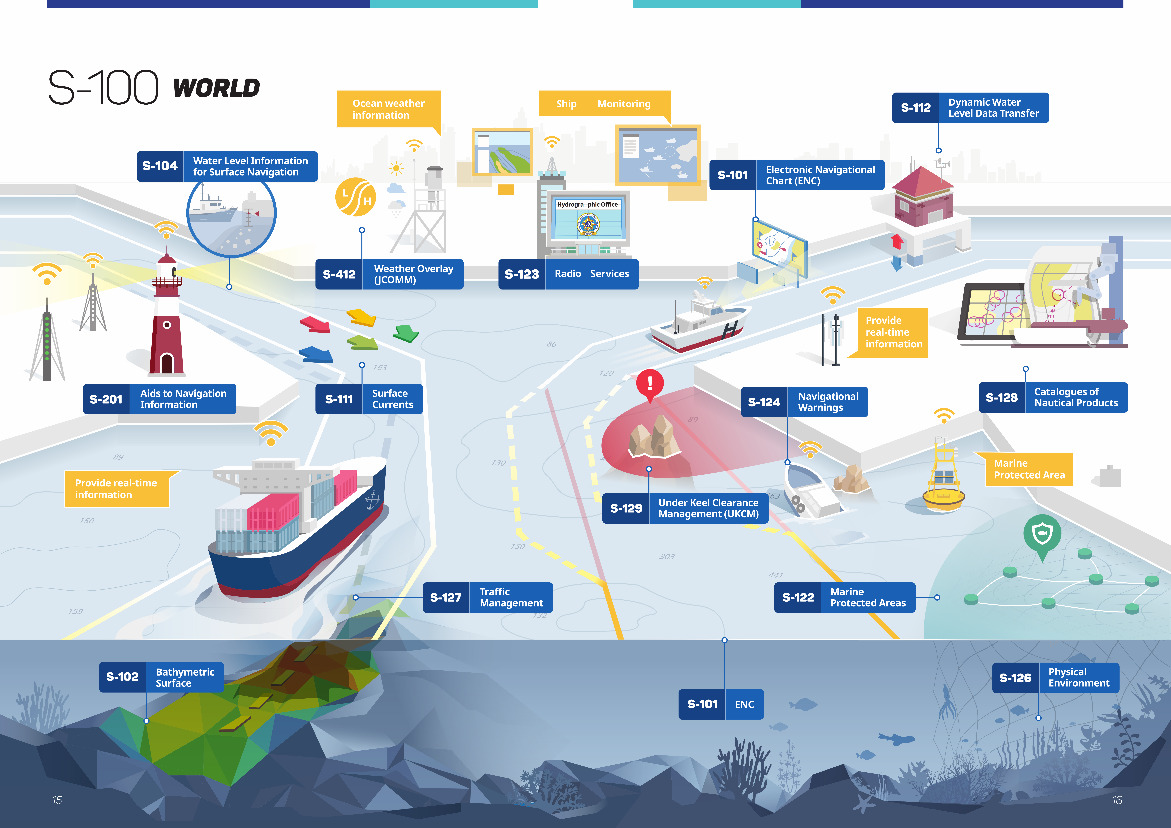
4 Digitalization as the major driver
The ongoing digitalization of all hydrographic processes, starting with sensor technology and continuing with the collection, transmission, processing and dissemination of hydrographic information, will provide data volumes of unprecedented scope and quality. What is still missing is a digital ecosystem to host the diversity of marine data to enable sovereign and sustained handling of such data stocks.
It is open at this stage if such an environment can be established under governmental supervision or if it will become an industry-driven commercial model. Maybe a combination of both approaches depending on the different socioeconomic paradigms in regions such as North America and Europe. The challenge for hydrographic services to contribute to such an ecosystem is the management of these data streams for their respective domain, the definition of data products derived from them according to the customer’s needs and their continuous quality-assured provision. The most striking visible change will come from the use of uncrewed instrument carriers (Fig. 7), which, in addition to their aforementioned benefits, contribute to the reduction of the carbon footprint of survey and inspection operations, support faster decision-making through real-time insights and ensure safer operations. Industry-wide scaling of these benefits of remote survey and inspection technologies will require a broader transformation in areas such as legislation, ways of working and obtaining new skills and competencies of all parties involved.
As ongoing digitalization is one side of the coin, communications will be the other one. As we know from our daily life, internet access has become the ’fifth element’”. If it becomes widely available at sea, this will definitely be a game changer. One of the most promising solutions is the Maritime Cloud: each naval asset will get its individual maritime identity, a globally accessible representation supported by cloud-based digital information technology. Under this concept, the ’ship’s avatar’ would contain static and operational information associated with the particular vessel and this would even apply to any sort of hydrographic information – predominantely ECDIS data fuel. Other features could include bridge windows that serve as augmented reality displays, identifying potential hazards that might otherwise be difficult to see, such as sea ice or tug boats (Fig. 8).
But whatever solution this whole process will finally present, there are two tendencies which seem clear. First, ECDIS as a stand-alone unit will probably disappear i.e. be absorbed as a function within an integrated environment embedded in a unified user interface. Secondly, more information will be given to machines that is presently confined only to humans. Consequently, it will allow machines to become even more involved in the navigational process. For instance, automated analysis taking into account the integrity of all data could readily merge positional and dynamic information with data that is already available digitally, such as ENCs, maritime safety information, meteorological and tidal predictions, etc. For decisions not involving other vessels, it potentially allows some very sophisticated decision-making processes regarding navigation to be automated. If such a trend materializes, visual presentation of chart items may generally be reduced to relevant information for the situation at hand. Visual detection remains particularly important and a key part of navigation on crewed vessels, enabling decisions to be made when there are other vessels in the vicinity. This is tied to the question as to whether humans will remain as the only detector and user of visual information for navigation purposes onboard vessels. Much research on the concept of uncrewed ships is being carried out nowadays. The relocation of all navigation competencies from ship to shore would see the end of the need to carry electronic charts as an on board device. However, without doubt, graphical presentation of maritime geoinformation will remain the most intuitive way of understanding our environment, whatever the future brings with new technologies and automation.
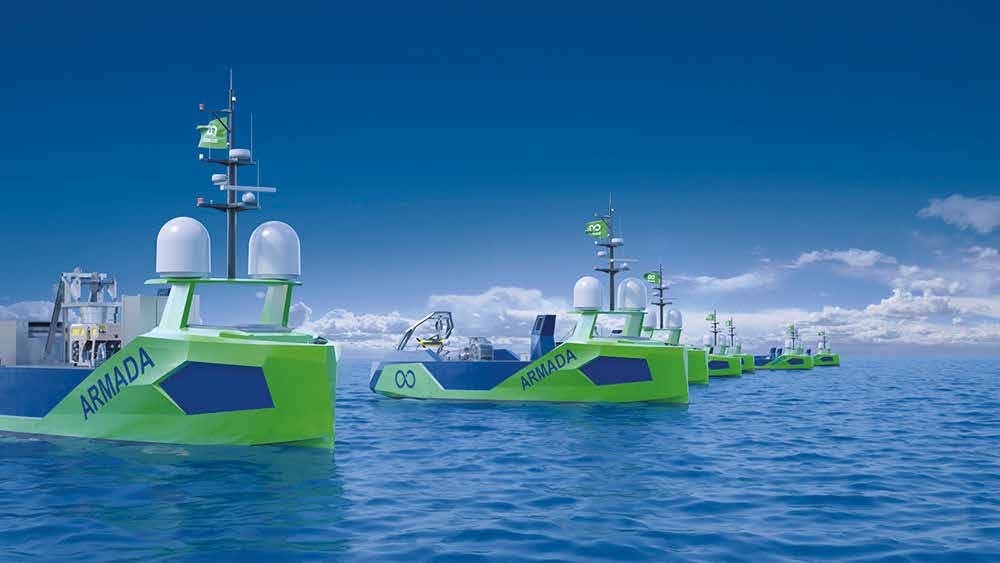
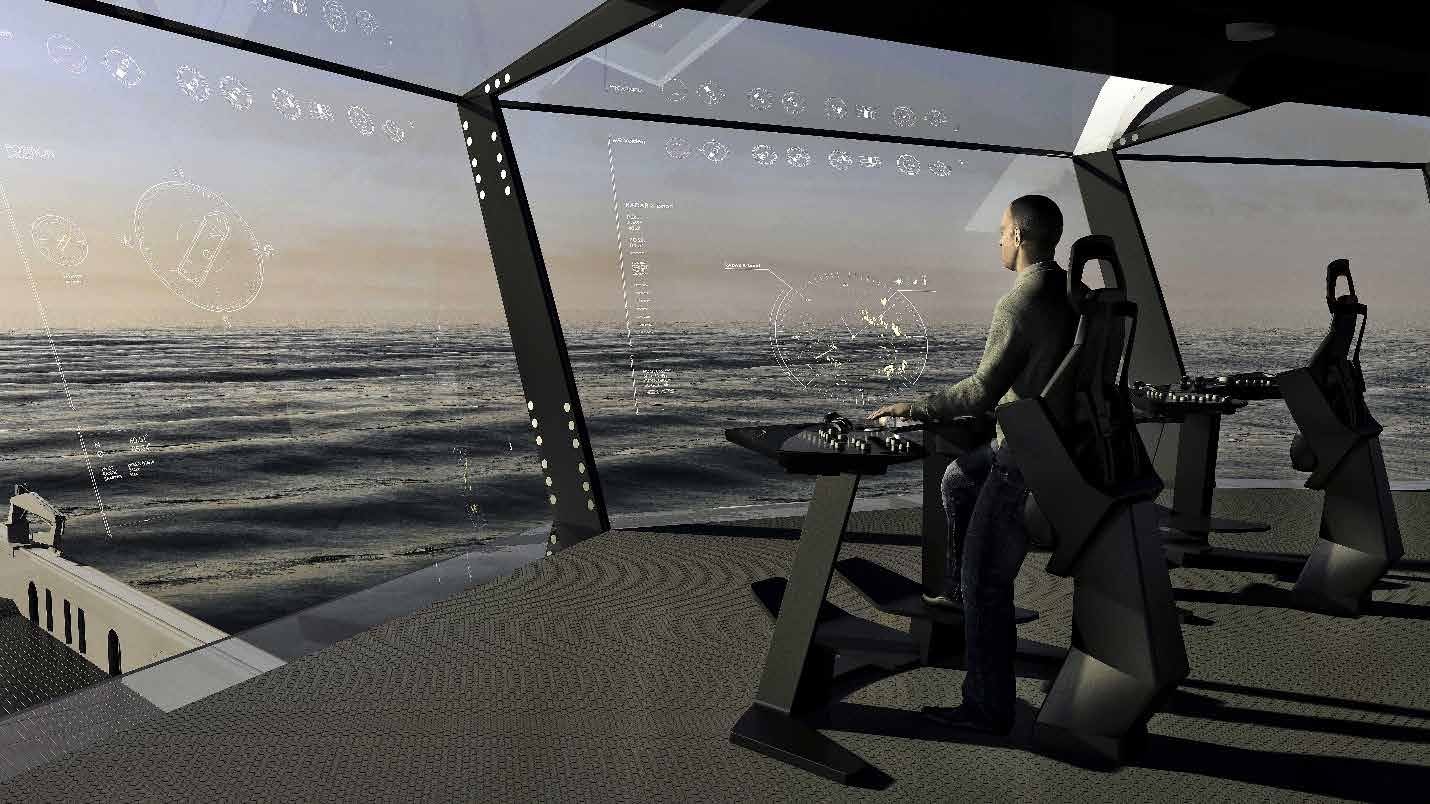
5 The human element
Coming back to the initial description of hydrography as an engineering science it is evident that the conduct of our discipline needs capable and motivated people. Ocean matters have always attracted younger people and there are numerous ocean literacy initiatives in place to support them. But what are the competencies of the “hydrographer of the future”? The Canadian Hydrographic Office convened an international workshop on this subject in April 2022.
The starting point was a discussion about the impact on hydrographic offices and the role of humans in this increasingly technology-driven environment. Will this person be predominantly a data manager and organizer or is there still a need for specific marine qualifications? The outcome of the discussion was that the ideal candidates had attended certified education courses and training as hydrographers or cartographers but there was also a tendency to hire staff with educational backgrounds in physical sciences, data science, IT and programming as well as GIS. These new recruits would need additional education & training in core hydrographic themes. This raises the question of the right balance between those two elements – one addressing the skill set and the other one the practice of skills application. Obviously, education is the core; training builds skills and helps hydrographers keep up with rapid advances in technology and hydrographic practices. Elements of marine spatial data infrastructures must be also incorporated as well as time at sea in order to create an informed understanding of hydrographic products in use. In many cases, hydrographers are “testers” for various hydrographic data collection devices as development is ongoing. The idea of “push button hydrographers” can be problematic as organizations are held accountable for the quality of collected data, and hydrographers need to have the skills and expertise required to ensure that the data collected are “good”; they need to understand the how and the why. In this context, it is important to understand that it is essential to evaluate and track the training and the experience of individuals within an organization. There is still a need for some hydrographers to be “jack of all trades”, able to carry out a variety of tasks, while others become increasingly specialized, and some aiming for a mix of both. In the end, hydrographic offices and industry are in need of talented employees who belong to either one of the three groups. The last group may be the best messengers to help increase knowledge of hydrography as a discipline among the broader population through outreach with educational institutions, show cool technology etc. This would help demonstrate the various career paths and retain talent. Mentorship through career development could help secure this long-term investment.
In order to get the best talent from around the world, we also need to increase diversity in the field. One area, which needs significant improvement, is gender balance in hydrography. The IHO has recently adopted this topic to its Work Programme under its Capacity Building strategy. Globally, the well-documented imbalance between the participation of women compared to men in the science, technology, engineering, and mathematics (STEM) fields as well as other maritime-related domains is also present in hydrography. This imbalance is apparent at all work levels but it is particularly evident at the more advanced career echelons and in governance and decision-making positions. The ambition to change this is relevant to hydrography because not only will it address issues of gender-equity, but it will open the field to a vast pool of talent that will potentially contribute new perspectives, skills, and creativity to the world of hydrographic sciences.
6 Outlook to the future
Surface navigation has seemingly been the traditional focus of hydrographic products for decades. However, recent estimates suggest that only 10% of all surveys are undertaken for the sole purpose to support nautical cartography. Other aspects related to the use and preservation of the marine environment have become crucial drivers. Therefore, an increased focus on coastal surveys, shallow waters below 10m, the continental shelf in areas far from navigation and the hitherto sparsely surveyed deep sea is to be expected. Despite new cost-effective uncrewed instrument carriers, data acquisition at sea remains complex. Therefore, it is important that the evaluation of measurement data is as comprehensive as possible for all recoverable information such as sediment, underwater habitats and unexploded ordnance. The key to this will be machine learning and artificial intelligence techniques.
The state of the oceans is a human issue. In order to provide the basis for sound political decisions, the engineering and scientific knowledge gained from the data must be translated into forms of communication that are understandable to the interested general public – and this is also true for hydrographic knowledge. It is not enough to limit oneself to the classic hydrographic repertoire of mapping the topography of the seabed and topics relevant to shipping. Spatial-temporal representations that interweave physical measurement data with data on biological and chemical processes of the sea are just as necessary as the expansion in the future of the systematic measurement tasks of hydrography to include maritime gravimetry, plastic particle pollution and underwater noise.
In view of this development, the relationship between hydrography and oceanography will naturally evolve. The measuring, monitoring part of ocean observation may increasingly be carried out under hydrography and be considered a routine government task. Oceanography will benefit from this flow of data delivered by hydrographers and will be able to focus on the analysis of the data streams and their interpretation, thereby gaining a better understanding of ocean processes.
Hydrography is active on a large scale in areas of the earth’s surface that either belong to the whole of humanity or are used internationally on a pro rata basis, for example by shipping. It is therefore of a dimension and importance which, for both technical and ethical reasons, can only be addressed as a coordinated mission. An indispensable prerequisite for this is political agreement, as provided by the major international treaties UNCLOS, SOLAS and MARPOL. Global initiatives under the umbrella of the UN, such as the Sustainable Development Goals and the Ocean Decade, address the need for action and trigger coordinated activities that ultimately amount to an expansion of the existing scope of regulation in favor of the conservation and responsible use of the seas and oceans. Coming back to my very first argument of this article: hydrography remains an engineering science but with a greatly expanded scope. Hydrography services a wealth of marine scientific and commercial disciplines. Hydrography is a laboratory for the use of cutting edge technology applied through cross-sectoral and cross-border collaboration. Hydrography is indispensable to gain enhanced marine knowledge, to understand better how this planet functions. An important instrument to make these insights productive is and remains the IHO which celebrated its 100th anniversary in 2021, a sign of its continued usefulness through the ages and capacity to adapt to changing times. The IHO, helps to create the technical standards and the personnel prerequisites in hydrography to help address humanity’s great challenges. In doing so the IHO delivers a best practice for the strength and benefits of international cooperation. Hydrographers of the world know: the future will be Blue and stand ready for a new voyage of Twenty Thousand Leagues Under the Sea.
Author’s biography
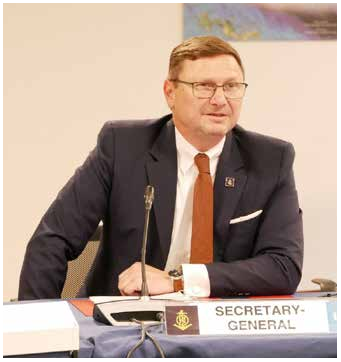
Dr Mathias Jonas is the Secretary-General of the International Hydrographic Organization (IHO) since 2017. Prior to this appointment he held the posts of Vice President of the Federal Maritime and Hydrographic Agency and National Hydrographer of Germany with responsibility for sea survey and sea cartography. Being a mariner, Dr Jonas has been involved in integrated navigation matters since the beginning of the nineties. In addition, he has completed the world’s first technical certification of an Electronic Chart Display and Information System in 1999 and has continuously contributed to IMO and IHO standardisation activities for navigation equipment, survey and cartography since. As one of the responsibilities of his current post, he holds the Chair of the Hydrographic Commission on Antarctica.
In the running period of Dr Jonas’ lead of the IHO Secretariat he managed to raise the profile of IHO as competent body in its key areas – technical standardisation and inter-regional cooperation – and succeeded also to widen this scope to all activities associated with the oceans, seas and navigable waters. His achievements in digitalization of the IHO Secretariats functions helped to keep IHO´s pace on the collaboration with Member States, relations with global partners and the working regime of all the IHO Committees and Working Groups despite the limitations induced by the COVID-19 pandemic.